To be sustainable, producers need to conserve energy while still maintaining or improving their facilities and the lives of the animals they house. This presentation was originally broadcast on January 19, 2024. Continue reading “Animal Feeding Operations Air Emission Estimation Methods”
Use of Vermifiltration as a Tool for Manure Management
This webinar discusses the science and economics behind the use of worms in the processes of composting (i.e., vermicomposting) and treatment of wastewater and manure liquid waste stream (vermifiltration). This presentation was originally broadcast on May 20, 2022. Continue reading “Use of Vermifiltration as a Tool for Manure Management”
Manure emissions during agitation and processing
Purpose
Recent deaths associated with hydrogen sulfide exposure from manure systems have highlighted the need for increased awareness to reduce health risks. While information on some aspects of hydrogen sulfide release from manure are available, there is limited information on the characteristics when agitating manure storages and in manure processing buildings that result in concentrations that are dangerous to human health. This project aimed to gather data on emissions from manure storages and processing to assess risks and develop mitigation strategies for these risks.
What Did We Do?
Our research team acquired over 20 days of field data (at multiple livestock farms) to assess the air concentrations from manure storages with and without agitation, for hydrogen sulfide, methane, ammonia, and particulate matter. The emissions were measured over the course of eight hours using numerous sets of sensors around the manure storage during agitation for each sampling event. Each sampling event had one backpack that was worn by a researcher with a set of sensors to represent the concentrations relevant to someone working in the area. Five additional sensor sets were placed around the manure storage. Some sensor sets remained in the same position throughout sampling (e.g., at the location of the agitation equipment controls) while others were moved around the storage. Researchers also measured the concentrations of these gases inside a manure processing room to assess the concentration changes with different air exchange rates. During each event manure samples were collected as well as weather data to relate to the manure emissions data.
What Have We Learned?
This research assessed the environmental and design conditions of manure systems that may lead to increased concentrations of gases that have human health implications. The results indicate critical operating parameters on how to manage manure systems to limit risk from gases produced from manure processing and storage areas. More details on the study results will be available soon and will be presented at the conference.
Future Plans
This information is also being integrated into an existing fact sheet, https://learningstore.extension.wisc.edu/collections/manure/products/reducing-risks-from-manure-storage-agitation-gases-p1865, to provide an updated resource which integrates this new data. This information will be shared in a variety of settings to increase awareness and guide practices to reduce health risks to those working with livestock manure.
Authors
Rebecca A. Larson, Associate Professor & Extension Specialist, Biological Systems Engineering, University of Wisconsin-Madison
Corresponding author email address
rebecca.larson@wisc.edu
Additional author
Anurag Mandalika, Assistant Professor, Audobon Sugar Institute, LSU AgCenter
Additional Information
Reducing Risks from Manure Storage Agitation Gases
Acknowledgements
This work is supported by Foundational Program CARE 2019-68008-29829 from the USDA National Institute of Food and Agriculture.
The authors are solely responsible for the content of these proceedings. The technical information does not necessarily reflect the official position of the sponsoring agencies or institutions represented by planning committee members, and inclusion and distribution herein does not constitute an endorsement of views expressed by the same. Printed materials included herein are not refereed publications. Citations should appear as follows. EXAMPLE: Authors. 2022. Title of presentation. Waste to Worth. Oregon, OH. April 18-22, 2022. URL of this page. Accessed on: today’s date.
How are Filth Flies Involved in Wasting Nitrogen?
Purpose
Filth flies are species from the Diptera order associated with animal feces and decomposing waste. Beef cattle raised on open pastures are especially susceptible to two species of filth flies: Face flies (Musca autumnalis De Geer) and Horn flies (Haematobia irritans (L.)) because these flies develop exclusively in fresh cattle manure. Filth fly impact on cattle health is related not only to the loss of body weight but also to the transmission of diseases like pink eye and mastitis (Basiel, 2020; Campbell, 1976; Hall, 1984; Nickerson et al., 1995).
Nitrogen losses from cattle’s manure has been reported for domestic flies (Musca domestica) and bottle flies (Neomyia cornicina) (Iwasa et al., 2015; Macqueen & Beirne, 1975). Despite the regular presence of face fly and horn fly in pastures, their effect on the nutrient cycles is little known. The purpose of this study is to understand the relationship between filth fly’s presence in cattle manure with the nitrogen losses caused by an increase in ammonia and nitrous oxide emissions.
What Did We Do?
The study was conducted in four pastures in the Georgia Piedmont: two near Watkinsville and two near Eatonton during June, July, and August of 2021. Ammonia volatilization and nitrous oxide emissions were measured on days 1, 4, 8, and 15 following dung deposition. Manure samples were collected on days 1 and 15. A static chamber was sealed for 24 h on each sampling date to capture manure’s ammonia and nitrous oxide emissions. In each chamber, a glass jar with boric acid was used to trap ammonia, and gas samples were collected. The gas samples were analyzed for nitrous oxide with a Varian Star 3600 CX Gas Chromatograph using an electron capture detector.
The number of filth flies was determined using a net trap covered by a black cloth that was set after 1 min of manure deposition, allowing the flies to oviposit for 10 min. On the days in which ammonia was not measured, a net trap was set to avoid additional oviposits, and record the emergence of filth flies. On the 15th day, we collected the filth flies that emerged from the eggs deposited in the manure during the first day.
What Have We Learned?
We found that cattle’s manure nitrogen loss as nitrous oxide (N2O) and ammonia (NH3) emissions have a direct relationship with the number of horn flies and face flies in the dung, Figure 1. Eighty percent of the flies trapped were horn flies. Dung with less than 5 flies can emit as little as 0.11 mg of N/kg of manure per day, while cattle manure with more than 30 flies can increase this emission by more than 10 times.

Every extra filth fly in manure can increase N emissions by 0.03 mg per kg of manure per day. According to NRCS, 59.1 lbs. of fresh manure is produced by a cow (approx. 1 000 pounds animal) every day (NRCS, 1995). Considering an average of 85 % relative humidity, 4.03 kg of dry manure can be produced per cow day. The actual economic threshold for horn fly is 200 flies per animal (Hogsette et al., 1991; Schreiber et al., 1987), considering a 1 to 1 sex ratio during emergence (Macqueen & Doube, 1988) we are assuming 100 female flies. Since the capacity of horn flies is 8-13 eggs per day (Lysyk, 1999), 100 female horn flies can generate approximately 1,000 new flies every day. Calculating the nitrogen emissions (4.03 kg of dry manure X 0.03 mg N kg manure x 1,000 flies per day) results in 121 mg of N loss per cow per day when assuming the number of flies is just at the economic threshold. In January of 2022, USDA released the Southern Region Cattle Inventory with a total of 91.9 million head, from which 30.1 million were beef cows (USDA, 2022). Considering the earlier numbers, the horn fly presence in the beef cattle of the Southern Region could be emitting 3,639 kg of Nitrogen to the atmosphere every day.
Future Plans
We will continue the study on ammonia and nitrous oxide emissions under the same conditions during another year to confirm the trends and accuracy of the results. Also, we will implement a study to analyze the effect of the introduction of a parasitic wasp Spalangia endius as a biological control on horn fly and face fly populations and therefore on the manure’s nitrogen losses.
Authors
Presenting author
Natalia B. Espinoza, Research Assistant, Department of Crop and Soil Science, University of Georgia
Corresponding author
Dr. Dorcas H. Franklin, Professor, Department of Crop and Soil Sciences, University of Georgia
Corresponding author email address
dfrankln@uga.edu or dory.franklin@uga.edu
Additional authors
Anish Subedi, Research Assistant, Department of Crop and Soil Science, University of Georgia
Dr. Miguel Cabrera, Professor, Department of Crop and Soil Sciences, University of Georgia
Dr. Nancy Hinkle, Professor, Department of Entomology, University of Georgia
Dr. S. Lawton Stewart, Professor, Department of Animal and Dairy Science, University of Georgia
Additional Information
Basiel, B. (2020). Genomic Evaluation of Horn Fly Resistance and Phenotypes of Cholesterol Deficiency Carriers in Holstein Cattle [PennState University]. Electronic Theses and Dissertations for Graduate Students.
Campbell, J. B. (1976). Effect of Horn Fly Control on Cows as Expressed by Increased Weaning Weights of Calves. Journal of Economic Entomology, 69(6), 711-712. https://doi.org/DOI 10.1093/jee/69.6.711
Hall, R. D. (1984). Relationship of the Face Fly (Diptera, Muscidae) to Pinkeye in Cattle – a Review and Synthesis of the Relevant Literature. Journal of Medical Entomology, 21(4), 361-365. https://doi.org/DOI 10.1093/jmedent/21.4.361
Hogsette, J. A., Prichard, D. L., & Ruff, J. P. (1991). Economic-Effects of Horn Fly (Diptera, Muscidae) Populations on Beef-Cattle Exposed to 3 Pesticide Treatment Regimes. Journal of Economic Entomology, 84(4), 1270-1274. https://doi.org/DOI 10.1093/jee/84.4.1270
Iwasa, M., Moki, Y., & Takahashi, J. (2015). Effects of the Activity of Coprophagous Insects on Greenhouse Gas Emissions from Cattle Dung Pats and Changes in Amounts of Nitrogen, Carbon, and Energy. Environmental Entomology, 44(1), 106-113. https://doi.org/10.1093/ee/nvu023
Lysyk, T. J. (1999). Effect of temperature on time to eclosion and spring emergence of postdiapausing horn flies (Diptera : Muscidae). Environmental Entomology, 28(3), 387-397. https://doi.org/DOI 10.1093/ee/28.3.387
Macqueen, A., & Beirne, B. P. (1975). Influence of Some Dipterous Larvae on Nitrogen Loss from Cattle Dung. Environmental Entomology, 4(6), 868-870. https://doi.org/DOI 10.1093/ee/4.6.868
Macqueen, A., & Doube, B. M. (1988). Emergence, Host-Finding and Longevity of Adult Haematobia-Irritans-Exigua Demeijere (Diptera, Muscidae). Journal of the Australian Entomological Society, 27, 167-174. <Go to ISI>://WOS:A1988P906100002
Nickerson, S. C., Owens, W. E., & Boddie, R. L. (1995). Symposium – Mastitis in Dairy Heifers – Mastitis in Dairy Heifers – Initial Studies on Prevalence and Control. Journal of Dairy Science, 78(7), 1607-1618. https://doi.org/DOI 10.3168/jds.S0022-0302(95)76785-6
NRCS, N. R. C. S. (1995). Animal Manure Management. RCA Publication Archive(7). https://www.nrcs.usda.gov/wps/portal/nrcs/detail/null/?cid=nrcs143_014211
Schreiber, E. T., Campbell, J. B., Kunz, S. E., Clanton, D. C., & Hudson, D. B. (1987). Effects of Horn Fly (Diptera, Muscidae) Control on Cows and Gastrointestinal Worm (Nematode, Trichostrongylidae) Treatment for Calves on Cow and Calf Weight Gains. Journal of Economic Entomology, 80(2), 451-454. https://doi.org/DOI 10.1093/jee/80.2.451
USDA. (2022). Southern Region News Release Cattle Inventory. https://www.nass.usda.gov/Statistics_by_State/Regional_Office/Southern/includes/Publications/Livestock_Releases/Cattle_Inventory/Cattle2022.pdf
A mass balance approach to estimate methane and ammonia emissions from non-ruminant livestock barns
Purpose
Producers are under pressure to demonstrate and document environmental sustainability. Responding to these pressures requires measurements to demonstrate greenhouse gas (GHG) emissions and/or changes over time. Stored manure emissions are a critical piece of livestock agriculture’s contribution to GHG production. Manure sample‐based estimates show promise for estimating methane (CH4) production rates from stored manure but deserve more extensive testing and comparison to farm‐level measurements. Understanding the causes for variability offer opportunity for more realistic and farm‐specific GHG emissions. Improved GHG measurements or estimates will more accurately predict current GHG emission levels, identify mitigation techniques, and focus resources where they are needed. This project offers an innovative approach to improvement of air quality and strengthens engagement by the livestock sector in sustainability discussions.
Although CH4 and ammonia (NH3) emissions from non-ruminant livestock production systems are primarily released from stored manure, current emission inventories (models) do not account for all production and management systems. The purpose of this project was to track flows of nitrogen, volatile solids (VS), and ash into and out of several commercial livestock barns to estimate CH4 and NH3 emissions. Using a mass balance approach, volatile components like nitrogen and volatile solids are supported through simultaneous balances with ash (fixed solids). These mass-balance based estimates can be compared to national inventory emission estimates and serve as sustainability metrics, regulatory reporting, and management decisions.
What Did We Do?
In the initial step of this project, experimental data for VS, the precursor to methane, are compared to fixed estimates in methane emission estimation tools, like the EPA State Greenhouse Gas Inventory Tool (US EPA, 2017).
The litter from a commercial turkey finishing barn housing between 13,000 and 18,000 birds was sampled weekly for one month, with one additional sampling day one month later. VS concentrations were analyzed for each sample and used to estimate total VS production per year assuming six 15,000 bird flocks (Soriano et al., 2022). A range of VS percentage values for deep-pit cattle facilities were taken from Cortus et al. (2021) and converted to total VS production per year. A range of VS concentrations for deep-pit swine manure storage were taken from Andersen et al. (2015) and used to find total VS production per year of that system as well. Next, total VS productions per year were estimated for the same three systems using the State Greenhouse Gas Inventory Tool.
What Have We Learned?
Table 1 summarizes all calculated total VS values and CH4 estimates per year for both the estimation tool and the experimental data. For each of the three systems, the state inventory estimated total VS value falls within the ranges calculated with experimental data, however, the estimates cannot account for the variabilities found within each system. As seen in the experimental total VS values, there can be a large range of VS production due to differences within specific operations of each system. Total VS relates directly to CH4 emissions, so accurate estimates are important for determining greenhouse gas emission potential of a specific operation.
Total VS production (kg/yr) | Emissions* | ||
---|---|---|---|
State Inventory | Experimental Values | m3CH4 | |
Feedlot Steer (500 head) | 334,990 | 260,758 – 1,002,675 | 1,262** |
Grower-Finisher Swine (1,200 head | 160,408 | 107,514 – 216,669 | 19,050 |
Turkey (15,000 head) | 314,594 | 206,838 – 359,245 | 1,699 |
*Emissions estimates found through the State Greenhouse Gas Inventory Tool **Feedlot steer emission estimate assumes an open feedlot manure management system |
Future Plans
Next steps for this study will include manure sampling at additional commercial turkey barns, deep-pit grower-finisher swine barns, and dairy cattle systems. Similar mass balances will be performed to determine total VS and nitrogen content to calculate CH4 and NH3 emissions from each system. These calculated values will again be compared to outputs of emission estimating tools.
Authors
Anna Warmka, Undergraduate Student, University of Minnesota – Twin Cities, Department of Bioproducts and Biosystems Engineering
Corresponding author email address
warmk011@umn.edu
Additional authors
Erin Cortus, Associate Professor, University of Minnesota – Twin Cities, Department of Bioproducts and Biosystems Engineering
Noelle Soriano, MS Student, University of Minnesota – Twin Cities, Department of Bioproducts and Biosystems Engineering
Melissa Wilson, Assistant Professor, University of Minnesota – Twin Cities, Department of Soil, Water, and Climate
Bo Hu, Professor, University of Minnesota – Twin Cities, Department of Bioproducts and Biosystems Engineering
Additional Information
Andersen, D.S., M.B. Van Weelden, S.L. Trabue, and L.M. Pepple. “Lab-Assay for Estimating Methane Emissions from Deep-Pit Swine Manure Storages.” Journal of Environmental Management 159 (August 2015): 18–26. https://doi.org/10.1016/j.jenvman.2015.05.003.
Cortus, E.L., B.P. Hetchler, M.J. Spiehs, and W.C. Rusche. “Environmental Conditions and Gas Concentrations in Deep-Pit Finishing Cattle Facilities: A Descriptive Study.” Transactions of the ASABE 64, no. 1 (2021): 31–48. https://doi.org/10.13031/trans.14040.
US EPA, OAR. “State Inventory and Projection Tool.” Data and Tools, June 30, 2017. https://www.epa.gov/statelocalenergy/state-inventory-and-projection-tool.
Soriano, N.C., A.M. Warmka, E.L. Cortus, M.L. Wilson, B. Hu, K.A. Janni. “A mass balance approach to estimate ammonia and methane emissions from a commercial turkey barn.” unpublished (2022).
Acknowledgements
This research was supported by the Rapid Agricultural Response Fund. We also express appreciation to farmer cooperators who allowed us to collect data on their farms and shared their observations with us.
Gaseous Emissions from In-house Broiler Litter
Purpose
Broiler litter is a valuable fertilizer but can also be a source of odorous and GHG emissions during production, storage, and land application. Impacts of these emissions are felt by local communities, posing respiratory health impacts and decreased quality of life, as well as increased deposition into soil and water systems. This study seeks to quantify the magnitude of emissions associated with in-house broiler litter and estimate variability across farms. Finally, the study evaluates litter parameters, such as litter age and chemical composition, for gas emission predictors.
What Did We Do?
A set of five active broiler houses in North Carolina were sampled to measure gaseous emissions (NH3, H2S, CH4, N2O, CO2, and VOCs) using headspace flux measurement gas samples. Headspace gas concentrations were measured at 1 hour and 3 hours after incubation at 30°C using a photoacoustic analyzer (Innova 1412) for NH3, CH4, N2O, and CO2 and Jerome 631-X was used to measure H2S, concentration. The headspace was also sampled to quantify VOCs associated with odorous emissions. After incubation, water extraction was used to quantify less volatile organic species that are associated with odorous emissions in the litter. Experimental setup is described in Figure 1. Statistical software, JMP, was utilized for analysis of litter composition on NH3, H2S, CH4, N2O, CO2, and VOC gaseous emissions.

What Have We Learned?
H2S emissions were very low (< 0.01 ppm) and did not produce statistically significant observations. There was a wide range of emissions from the litter samples for different gases as shown in Figure 2: 146-555 ppm NH3, 1.5-22 ppm N2O, 4,077-50,835 ppm CO2, and 9.1-43.3 ppm CH4. The differences between farms accounted for 86%, 81%, 76%, and 84% of the variability in NH3, N2O, CO2, CH4 observations, respectively. This could be attributed to differences in integrator and management strategies. Moisture content and age of the litter were the primary contributing factors to increased gaseous emissions from all samples. More specifically, NH3 was largely impacted by pH (p < 0.01), while N2O, CO2, and CH4 were largely impacted by C:N (p < 0.01). Quantitative VOC analysis was difficult due to the number of gases detected by the GC-MS (20+), however the most common species present in the litter samples were a variety of volatile fatty acids, alcohols, phenol, as well as a few amines, ketols, and terpenes.

Future Plans
These results will serve as baseline emission readings for odor and emission control strategies. We are currently developing Miscanthus-derived biochar as a poultry litter amendment for emission mitigation in poultry houses. This dataset will inform our decision making to help target gaseous species of top concern in NC broiler litter by methods of physical and chemical biochar modification.
Authors
Presenting author
Carly Graves, Graduate Research Assistant, North Carolina State University
Corresponding author
Dr. Mahmoud Sharara, Assistant Professor & Waste Management Extension Specialist, North Carolina State University
Corresponding author email address
msharar@ncsu.edu
Acknowledgements
Funding for this project is through Bioenergy Research Initiative (BRI)- NC Department of Agriculture and Consumer Services (NCDA&CS): Miscanthus Biochar Potential as A Poultry Litter Amendment
Emissions from Composting
This webinar focuses on air emissions associated different methods of composting and the subsequent emissions when composted manure is applied to cropland. This presentation was originally broadcast on September 18, 2020. More… Continue reading “Emissions from Composting”
Recent Research Efforts to Reduce Greenhouse Gas Emissions
This webinar discusses research projects focused on reducing greenhouse gases, odorous volatile organic compounds (VOCs), ammonia, and hydrogen sulfide. This presentation was originally broadcast on June 21, 2019. More… Continue reading “Recent Research Efforts to Reduce Greenhouse Gas Emissions”
Evaluation of current products for use in deep pit swine manure storage structures for mitigation of odors and reduction of NH3, H2S, and VOC emissions from stored swine manure
The main purpose of this research project is an evaluation of the current products available in the open marketplace for using in deep pit swine manure structure as to their effectiveness in mitigation of odors and reduction of hydrogen sulfide (H2S), ammonia (NH3), 11 odorous volatile organic compounds (VOCs) and greenhouse gas (CO2, methane and nitrous oxide) emissions from stored swine manure. At the end of each trial, hydrogen sulfide and ammonia concentrations are measured during and immediately after the manure agitation process to simulate pump-out conditions. In addition, pit manure additives are tested for their impact on manure properties including solids content and microbial community.
What Did We Do?

We are using 15 reactors simulating swine manure storage (Figure 1) filled with fresh swine manure (outsourced from 3 different farms) to test simultaneously four manure additive products using manufacturer recommended dose for each product. Each product is tested in 3 identical dosages and storage conditions. The testing period starts on Day 0 (application of product following the recommended dosage by manufacturer) with weekly additions of manure from the same type of farm. The headspace ventilation of manure storage is identical and controlled to match pit manure storage conditions. Gas and odor samples from manure headspace are collected weekly. Hydrogen sulfide and ammonia concentrations are measured in real time with portable meters (both are calibrated with high precision standard gases). Headspace samples for greenhouse gases are collected with a syringe and vials, and analyzed with a gas chromatograph calibrated for CO2, methane and nitrous oxide. Volatile organic compounds are collected with solid-phase microextraction probes and analyzed with a gas chromatography-mass spectrometry (Atmospheric Environment 150 (2017) 313-321). Odor samples are collected in 10 L Tedlar bags and analyzed using the olfactometer with triangular forced-choice method (Chemosphere, 221 (2019) 787-783). To agitate the manure for pump-out simulation, top and bottom ‘Manure Sampling Ports’ (Figure 1) are connected to a liquid pump and cycling for 5 min. Manure samples are collected at the start and end of the trial and are analyzed for nitrogen content and bacterial populations.
The effectiveness of the product efficacy to mitigate emissions is estimated by comparing gas and odor emissions from the treated and untreated manure (control). The mixed linear model is used to analyze the data for statistical significance.
What we have learned?
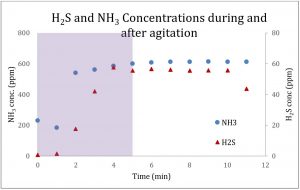
U.S. pork industry will have science-based, objectively tested information on odor and gas mitigation products. The industry does not need to waste precious resources on products with unproven or questionable performance record. This work addresses the question of odor emissions holistically by focusing on what changes that are occurring over time in the odor/odorants being emitted and how does the tested additive alter manure properties including the microbial community. Additionally, we tested the hydrogen sulfide and ammonia emissions during the agitation process simulating pump-out conditions. For both gases, the emissions increased significantly as shown in Figure 2. The Midwest is an ideal location for swine production facilities as the large expanse of crop production requires large fertilizer inputs, which allows manure to be valued as a fertilizer and recycled and used to support crop production.
Future Plans
We develop and test sustainable technologies for mitigation of odor and gaseous emissions from livestock operations. This involves lab-, pilot-, and farm-scale testing. We are pursuing advanced oxidation (UV light, ozone, plant-based peroxidase) and biochar-based technologies.
Authors
Baitong Chen, M.S. student, Iowa State University
Jacek A. Koziel*, Prof., Iowa State University (koziel@iastate.edu)
Daniel S. Andersen, Assoc. Prof., Iowa State University
David B. Parker, Ph.D., P.E., USDA-ARS-Bushland
Additional Information
- Heber et al., Laboratory Testing of Commercial Manure Additives for Swine Odor Control. 2001.
- Lemay, S., Stinson, R., Chenard, L., and Barber, M. Comparative Effectiveness of Five Manure Pit Additives. Prairie Swine Centre and the University of Saskatchewan.
- 2017 update – Air Quality Laboratory & Olfactometry Laboratory Equipment – Koziel’s Lab. doi: 10.13140/RG.2.2.29681.99688.
- Maurer, D., J.A. Koziel. 2019. On-farm pilot-scale testing of black ultraviolet light and photocatalytic coating for mitigation of odor, odorous VOCs, and greenhouse gases. Chemosphere, 221, 778-784; doi: 10.1016/j.chemosphere.2019.01.086.
- Maurer, D.L, A. Bragdon, B. Short, H.K. Ahn, J.A. Koziel. 2018. Improving environmental odor measurements: comparison of lab-based standard method and portable odour measurement technology. Archives of Environmental Protection, 44(2), 100-107. doi: 10.24425/119699.
- Maurer, D., J.A. Koziel, K. Bruning, D.B. Parker. 2017. Farm-scale testing of soybean peroxidase and calcium peroxide for surficial swine manure treatment and mitigation of odorous VOCs, ammonia, hydrogen sulfide emissions. Atmospheric Environment, 166, 467-478. doi: 10.1016/j.atmosenv.2017.07.048.
- Maurer, D., J.A. Koziel, J.D. Harmon, S.J. Hoff, A.M. Rieck-Hinz, D.S Andersen. 2016. Summary of performance data for technologies to control gaseous, odor, and particulate emissions from livestock operations: Air Management Practices Assessment Tool (AMPAT). Data in Brief, 7, 1413-1429. doi: 10.1016/j.dib.2016.03.070.
Acknowledgments
We are thankful to (1) National Pork Board and Indiana Pork for funding this project (NBP-17-158), (2) cooperating farms for donating swine manure and (3) manufacturers for providing products for testing. We are also thankful to coworkers in Dr. Koziel’s Olfactometry Laboratory and Air Quality Laboratory, especially Dr. Chumki Banik, Hantian Ma, Zhanibek Meiirkhanuly, Lizbeth Plaza-Torres, Jisoo Wi, Myeongseong Lee, Lance Bormann, and Prof. Andrzej Bialowiec.
The authors are solely responsible for the content of these proceedings. The technical information does not necessarily reflect the official position of the sponsoring agencies or institutions represented by planning committee members, and inclusion and distribution herein does not constitute an endorsement of views expressed by the same. Printed materials included herein are not refereed publications. Citations should appear as follows. EXAMPLE: Authors. 2019. Title of presentation. Waste to Worth. Minneapolis, MN. April 22-26, 2019. URL of this page. Accessed on: today’s date.
Performance of Mitigation Measures in the Dairy Sector under Future Climate Change
Proceedings Home | W2W Home 
Purpose
Climate change is an economic, environmental and social threat, and worthy of scientific study. Immediate action must be taken to reduce greenhouse gas emissions and mitigate negative impacts of future climate change. Proposed action can start at the farm level and has the potential of making a contribution to mitigation of climate change. Dairy farmers are able to significantly reduce their emissions by implementing better management practices, primarily through feed production, enteric fermentation, and manure management. We model the corresponding changes in emissions from proposed mitigation efforts to understand their impact on global climate change.
What did we do?
Best Management Practices (BMPs) for dairy systems have been identified and simulated using the Integrated Farm System Model (IFSM). Simulations representative of a large New York farm (1500 cows) and a small Wisconsin farm (150 cows) estimated the emission of greenhouse gases for a whole farm system. Percent reductions were calculated by comparing a baseline scenario without any implemented mitigation, to scenarios that included the identified BMPs. Refer to Table 1 for emission and percent reduction estimates for the simulated BMPs.
Percent reduction estimates were then applied to a projected “business as usual” emission scenario. This scenario prescribes anthropogenic emissions through 2100 and excludes any climate action or policy after 2015. Taking 2020 as a reference year and 2050 as a target year, we applied the estimated percent reductions to the projected global agricultural emissions. Emission reductions were decreased linearly from 2020 to 2050, and held constant between 2050 and 2100 (Figure 1). This assumes that all farms globally can reduce emissions despite increases in production. To compare the performance of the mitigation measures under future climate change, we employed a fully coupled earth system model of intermediate complexity – the Integrated Global System Model (IGSM). The model includes an interactive carbon-cycle capable of addressing important feedbacks between the climate and terrestrial biosphere.
What have we learned?
Action taken globally in the agricultural sector to reduce greenhouse gas emissions over the first half of the 21st century is likely to have an impact in mitigating global warming. Following a “business as usual” emission scenario without any climate policy or action beyond 2015, an increase in global mean surface temperature by the end of the 21st century (2081-2100) relative to pre-industrial (1961-1990) levels is projected to be 2.8 C to 3.5 C (Figure 2). This exceeds the 2 C temperature target described as the maximum warming allowed to avoid dangerous and irreversible climate change. An associated net radiative
forcing for the “business as usual” scenario is projected to be 7.4 W/m^2 by 2100 (Figure 3). Adopting the identified BMPs in the dairy sector and decreasing global agricultural emissions by 2050 is projected to decrease global mean surface temperatures for 2100 by 0.2 C and net radiative forcing by 0.4 W/! m^2 on av erage. In summary, this modeled experiment demonstrates that ongoing efforts to decrease greenhouse gas emissions in the dairy and agricultural sector are effective at reducing the overall warming of climate change.
Future Plans
Future work will look further into the evolution of regional temperature and rainfall profiles for the mitigation scenarios. Then, ecological risk assessment methodologies will be applied to determine the probable impacts of climate change by each scenario on agricultural production.
Corresponding author, title, and affiliation
Kristina Rolph – Graduate Student, The Pennsylvania State University.
Corresponding author email
Other authors
Chris Forest – Associate Professor of Climate Dynamics, The Pennsylvania State University.
Rob Nicholas – Research Associate, Earth & Environmental Systems Institute.
Additional information
- The Sustainable Dairy Project, funded by the USDA, researches alternative management practices in the dairy industry. http://www.sustainabledairy.org
- The Integrated Farm System Model simulates all major farm components to represent the many biological and physical processes on a farm. https://www.ars.usda.gov/northeast-area/up-pa/pswmru/docs/integrated-farm-system-model/
- The MIT Integrated Global System Model is a fully coupled earth system model of intermediate complexity designed to analyze interactions between human activities and the Earth system. https://globalchange.mit.edu/research/research-tools/global-framework
Acknowledgements
This material is based upon work that is supported by the National Institute of Food and Agriculture, U.S. Department of Agriculture, under award number 2013-68002-20525. Any opinions, findings, conclusions, or recommendations expressed in this publication are those of the author(s) and do not necessarily reflect the view of the U.S. Department of Agriculture.